Current
Research on Molecular Processing
Radiation damage, a new
understanding
In the life sciences the role of
electron-driven processes is being recognized as crucial to our
understanding of radiation damage of cellular material. The mechanisms by
which such degradation occurs have been the subject of considerable research
effort with genotoxic effects of ionizing radiation in living cells being
commonly attributed to direct impact of high-energy quanta or by complex
radical chemistry (triggered by production of OH species by primary ionizing
radiation). However recently this explanation has recently been questioned
by the pioneering work of Sanche and co-workers who suggest that DNA lesions
are induced by the lower energy, secondary electrons generated by the
primary ionizing radiation. Data of Sanche and co-workers (figure3) revealed
that :
- low energy electron irradiation directly
induces both single and double strand breaks at energies well below the
ionization limit of DNA (7.5eV) and
- that the probability of strand breaks
are one to two orders of magnitude larger for electrons than for photons
of corresponding energy.
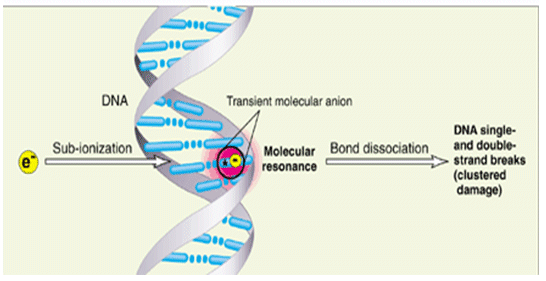
Figure 3: Most energy deposited in cells by ionizing
radiation is channelled into free secondary electrons with energies between
1 eV and 20 eV (B. Boudaïffa et al., Science 287 (2000) 1658)
The majority of the copious secondary electrons (~5 x 104 per
MeV) created within 10-15s along the radiation track have energies below 20
eV (Figure 4) . These low-energy electrons must undergo multiple inelastic
scattering events as they thermalise. The primary energy-loss channels for
electrons with energies typical of the secondary distribution are
ionization, direct electronic excitation and most important (but until
recently little studied) resonance scattering. The latter results in
the formation of Temporary Negative Ions (TNIs), which decay via electron
autodetachment and dissociative electron attachment (DEA) the latter process
leading to direct dissociation of the parent molecule as noted above. This
process may be summarised in the two step ‘reaction’;
e + M-ABC ---> (M-ABC) (1)
(M-ABC)- ---> M- ABC (2)
where (M-ABC)- is the temporary negative ion which decays to a
residual anion (M-) and a (reactive) molecular fragment ABC, for
example this may be the case in the STM experiment mentioned above where e +
C6H5I->C6H5 +I-.
 
Hence the process of DEA provides a direct low energy process for the
degradation of DNA (and other key cellular material) by resonant electron
attachment to basic molecular components (base, deoxyribose, phosphate, or
hydration H2O). Recent data suggest that single strand damage is site
specific and proceeds through discrete molecular bond rupture. This
in turn suggests that double strand breakage is simply induced through local
chemical reactivity. The DEA fragments produced within the cellular DNA
subsequently reacts with adjacent bases (at 3.4Å), or close-lying the
phosphate-sugar backbone (at < 5Å) leading to clustered damage within the
DNA strand.
Hence in contrast to traditional models of radiation chemistry which
are based upon statistical modelling it would appear that cellular damage
may be based upon single collisional phenomena involving transient molecular
resonances localised on DNA basic components.
In this project we wish to explore this hypothesis through an
intercomparison of the TNI formed from nucleotide bases and damage in DNA
(and RNA) during low energy electron irradiation.
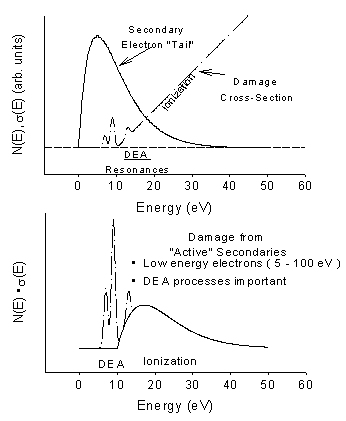
Figure 4: Top Frame: Typical energy distribution of
secondary electrons emitted during a primary ionizing event. Also shown are
the estimated relative cross-sections for some of the main inelastic
electron energy-loss channels that the secondaries undergo as they traverse
the interface. Lower Frame: Estimate of the effective “damage” probability
obtained by convoluting the energy-loss cross sections with the secondary
electron energy distribution.
|